DISCLAIMER
The information presented here is meant to be used as an educational asset for the Space community, both for commercial, non-commercial entities and individual actors; the use case has been generated through IENAI’s GO™, an intuitive thruster design and mission analysis tool on the cloud, which is open-access and free-to-use for the community. External data and assets have been gathered in good-will and, to the best of our knowledge, are accurate, but are not intended to replace official information released by companies, institutions, or other stakeholders. All author’s rights have been reserved; reproductions shown here are intended with educational uses only.
The present use case is intended to showcase the capabilities of IENAI’s ATHENA™ electrospray thrusters in an application for constellation deployment of Planet Labs’ Flocks. The ultra-high efficiency and uniquely scalable nature of the thruster allows for a reduced and customizable form factor, tailored to the requirements and limitations of the satellite platform and the specific maneuvers for which it is intended. This application has not been sponsored by Planet Labs in any way.
TL;DR
- Electric propulsion provides faster deployment and extended mission lifetime, and offers the potential of improving revisit rate and optical resolution.
- ATHENA™ thrusters reduce the deployment time of the entire constellation from about 300 days to just 12 days (including altitude reduction).
- The resolution provided by the Earth Observation constellation may be improved by lowering 60 km of altitude, while still performing station keeping for more than 3.5 years after deployment, considerably extending the lifetime of the mission.
- The propulsion system uses less than 130 grams of propellant for 3 different maneuvers: Orbit phasing, Orbit transfer and Station keeping.
→Download the summary for the Dove mission!
→Register for GO™ and create your custom thrusters!
MOTIVATION
Planet Labs holds the record for the largest, and perhaps most successful, constellation of nanosatellites currently in use: 439 Doves and SuperDoves, which make up the companies “flocks”, have been launched since 2013, with more than 150 operating today (the constellation is well into its “replenishment” phase), providing with Earth Observation capabilities of the Earth at under 4m/pixel resolution. The average lifetime of the Doves is about 2.5 years.
Typical launches send together tens of satellites (44 on the latest SpaceX Transporter 3 mission), which are deployed at close orbits and then require phasing in order to be uniformly spread around the Earth. In order to do this either propulsive maneuvers or differential drag can be employed; however, Doves are extremely packed (up to the tuna can!), as can be gathered from publicly available images, and therefore most propulsion systems on the market simply do not fit. Enpulsion’s NANO thruster flew in January 2018 in a 3U CubeSat Flock-3p from Planet occupying 0.8 U as publicly stated.
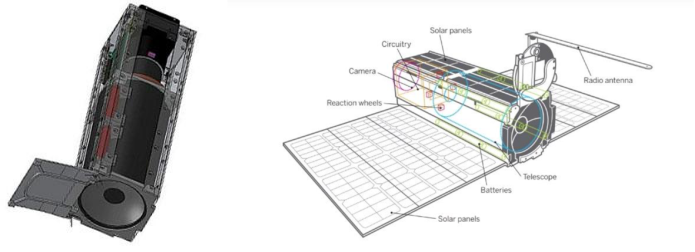
To date, Planet has employed differential drag in order to achieve True Anomaly phasing of their satellites. Foster et al. provides a methodology for the differential drag control of a large fleet of propulsion-less satellites deployed in the same orbit through a simple controller and two control modes: high drag (panels against the incoming flow) and low drag (panels parallel to the flow); the analysis was then compared to the real deployment of the Flock-2p batch of 12 satellites, launched into a circular 510 km sun-synchronous orbit in June 2016.
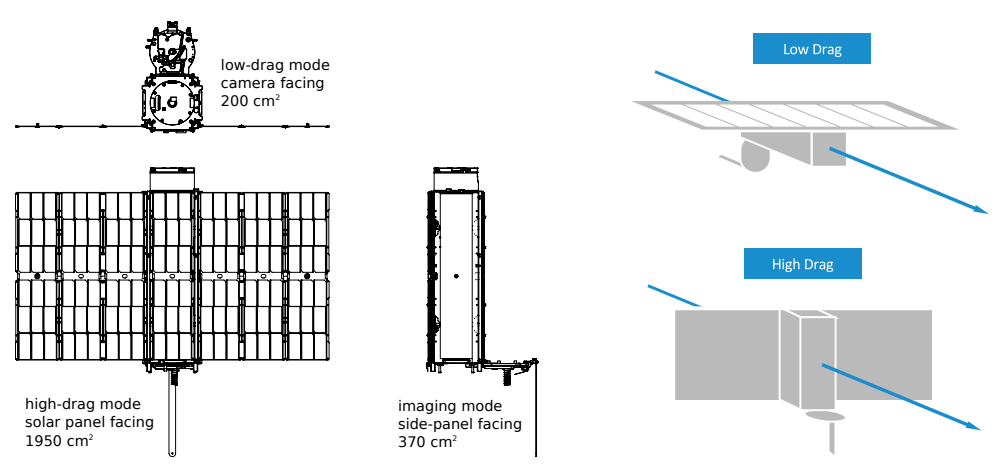
The “area-based” ratio of ballistic-coefficients should be around 10:1 for the high- and low-drag modes; however, Foster concludes that the actual is closer to 3:1 due to a combination of satellite duty-cycle effects (differential drag may only be performed outside of the satellite’s intended operation modes), skin friction effects and attitude pointing inaccuracies. Due to this, the phasing maneuver is completed in well over a year, with only 150 degree separation achieved with regards to the reference satellite in the first 100 days, potentially leading to a suboptimal geometry of the constellation batch and long gaps in between revisits, potentially heavily impacting the quality of the intended service.
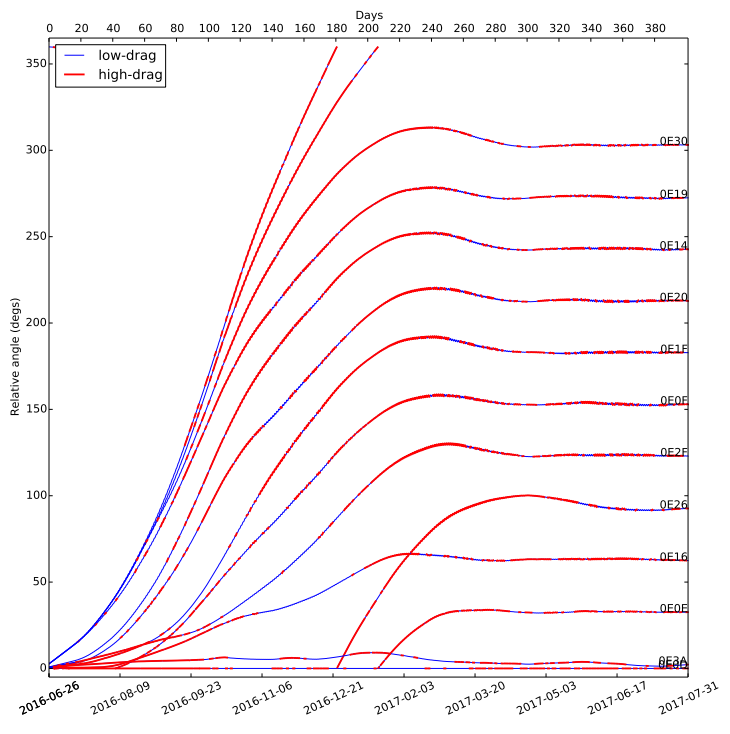
In order to avoid lengthy deployment times for a constellation such as Planet’s Flocks, improve the performance of its Earth Observation capabilities and provide longer operational lifetimes, this use case presents the “inverse propulsion problem”, as solved through IENAI’s GO™ tool, obtaining an optimal configuration of the ATHENA™ thruster for the mission’s requirements, during a theoretical preliminary design phase.
SIMULATING IN GO™
The mission presented can be easily translated into the required input parameters in GO™. The first step will be to define these parameters on the user-friendly Configuration view. This section allows the user to define the values for the platform, thruster envelope and initial orbit.
Parameter | Value |
---|---|
Platform | 3U |
Solar panels | Triple deployable |
Thruster Pointing | Off-Axis |
Maximum Power | 60 W |
Total Mass | 5 kg |
Launcher | PSLV by ISRO |
The configuration used for this mission is given in the table above. Platform, deployables and launcher are inputted to be as close to publicly released data from Planet as possible. Thruster pointing is an assumption made due to the characteristics of this mission: since ATHENA can’t be placed in any of the On-Axis faces due to the Dove’s payload and Tuna-can, we assume it would be placed perpendicular to the main axis of the platform. While a mid-drag mode could be exploited here, we assume the worst case scenario, the satellite being always in high-drag mode. A more detailed explanation is provided in the results section.
The 12 satellites compose an equally-spaced, coplanar constellation, this is a satellite placed every 30º of true anomaly. For this mission we are simulating the Doves in the worst case scenario in terms of ΔV which is the Dove placed at 180º from the injection. The hypothetical mission, maintaining the original launch, is divided into three main orbital maneuvers:
- In-plane phasing with RAAN compensation (deployment).
- Orbit transfer to 450 kms to improve resolution and revisit rate.
- Drag compensation for orbit maintenance.
Applying these maneuvers allows Doves to have faster deployment while improving resolution, revisit rate and mission lifetime, as will be discussed below. Note that the maneuvers for True Anomaly changes larger than 180º can be calculated in GO™ by choosing the required figure, but will take less than the 180º maneuver, since they are achieved by performing the opposite maneuver in terms of altitude change.
MISSION CONFIGURATION
The first step in GO™, once the mission is created, is to select the platform configuration, taken from the Flock-2p Doves: 3U platform with triple-deployable solar panels. The estimated maximum power production is about 60 W and the total mass of the Doves is reported to be about 5 kg. The thruster pointing is selected to be Off-Axis (high-drag mode), since it is the only possible configuration to add a thruster due to the current requirements of Flock 2p, with an estimated front area of 1950 cm2.
Once selected, the values of thruster pointing and thruster power are defined: we used a 9 W power budget for the thruster, assuming that said power would be available for a “propulsion mode” in the mission. Furthermore, as we will see in the results, this value will restrict the use of a single thruster module (on a 1U area), which is agreeable with the presumed lack of space in the platform.
Once these parameters are defined, we proceed to select the initial orbit. In this step, the user has two options: Custom orbit or Launcher selection. For this particular case, we used the custom orbit. Flock 2p was launched by a PSLV launcher from ISRO, the injection orbit used was 510 km of altitude and 98º of inclination. Once the orbit is captured, the configuration is saved by clicking on the “SAVE” button, after which we are redirected to the maneuvers scenario view.
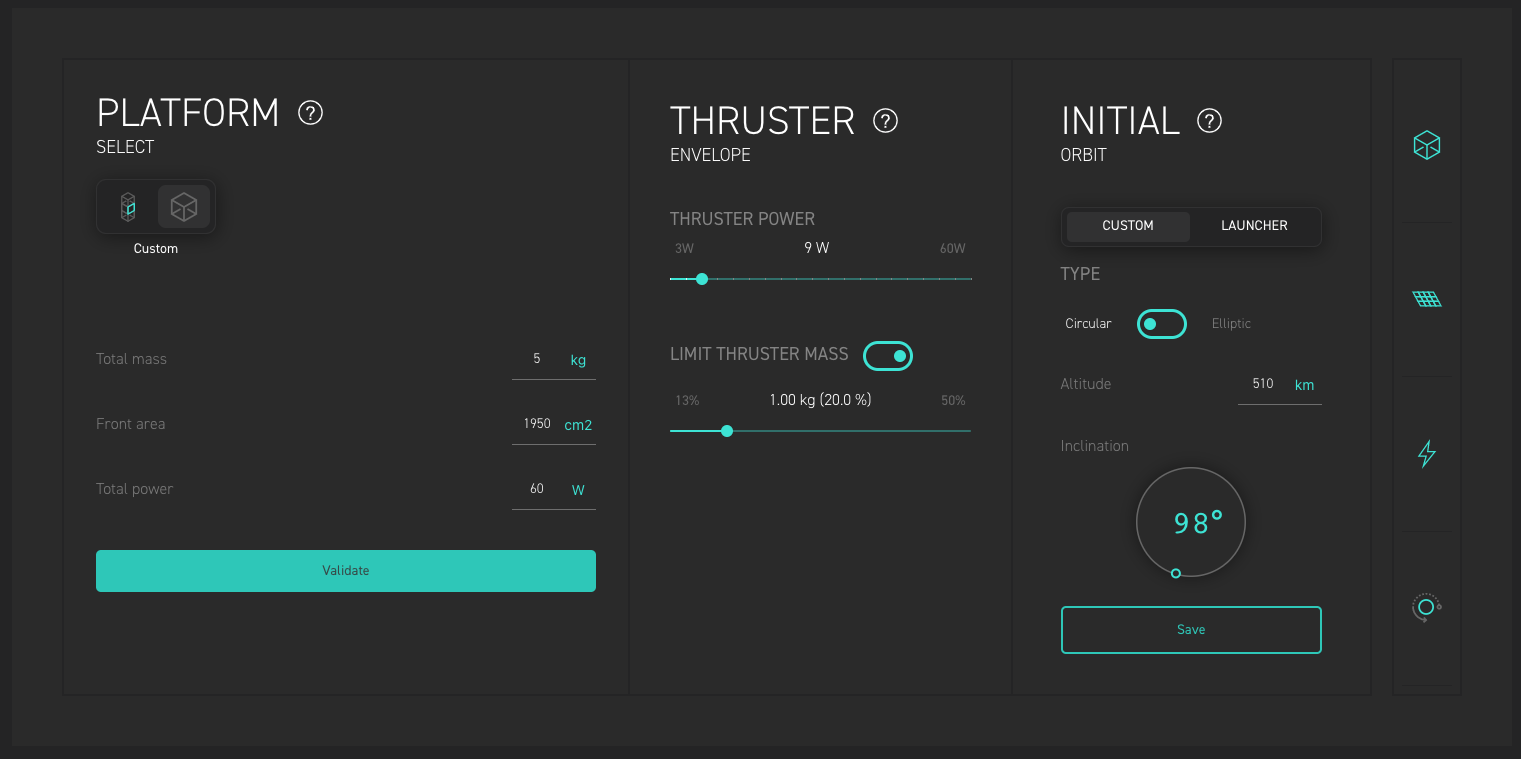
Orbit phasing
Once the initial configuration is saved, the next step is to sequentially add the maneuvers required throughout the mission. In the Maneuver Scenario view in GO™, we select “Orbit phasing” as the maneuver type and then set a 180º true anomaly shift; we toggle ON the RAAN shift compensation, since the semi-major axis change leading to the phase change generates a small RAAN difference, which can be balanced by adding a small out-of-plane thrust component, at the expense of slightly larger propellant consumption and longer maneuver times. Once these values are selected, the minimum duration is calculated automatically by GO™, for the shortest physically possible maneuver. In this case, we select the minimum duration, but the user can play with this value to allow for longer maneuvers at lower propellant costs. Clicking on “Calculate maneuver” shows the results and orbital element time profiles during the maneuver.
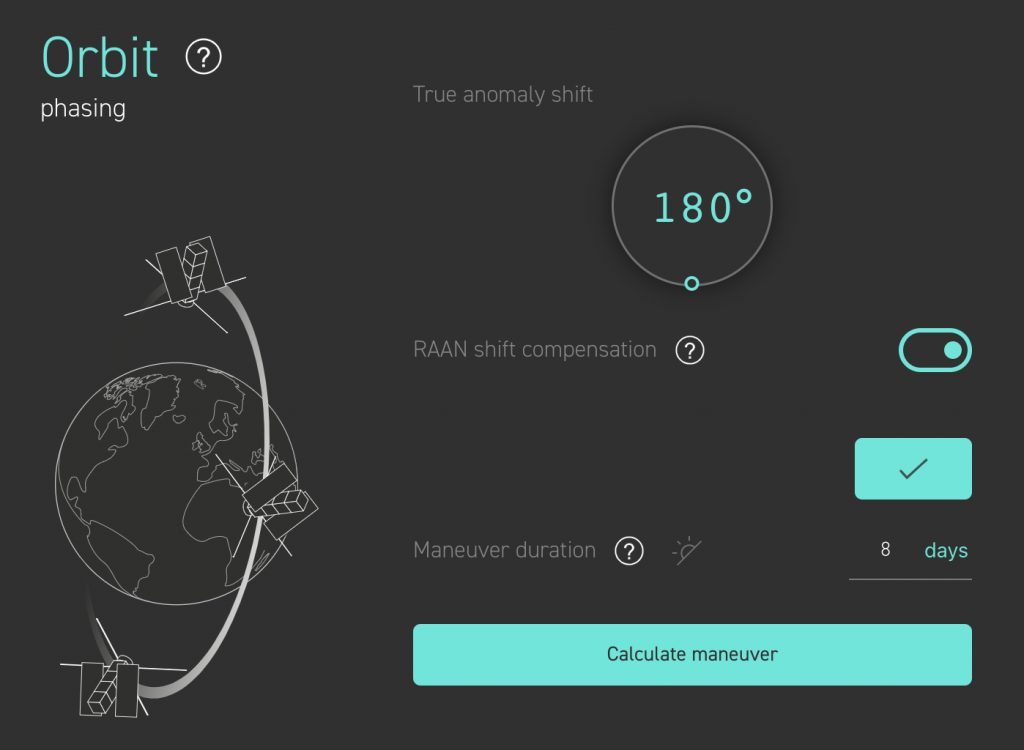
The resulting maneuver, shown in Fig. 6, spans 7.1 days, consuming 15.6 g of propellant. To reduce the deployment time and to be able to compensate the RAAN shift from Earth’s oblateness, continuous thrusting is performed at maximum power (9 W), thus firing 6 thruster heads simultaneously. The benefits of using on-board propulsion is obvious, reducing the phasing deployment time by a factor of 42 with respect to the differential drag approach, if the original altitude were to be maintained. The time saved is indicative of how the deployment rate capabilities may be improved through propulsive maneuvers, which would also allow fast replacement of non-functional satellites in the orbital plane, putting quick remedy to the suboptimal coverage in the event of a failure.
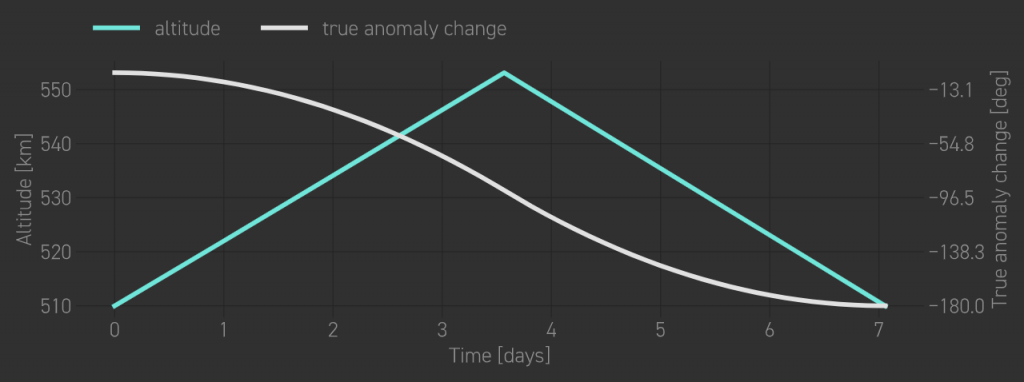
One of the additional advantages of using on-board propulsion is the ability to modify the orbit altitude over time. In 2019, Planet lowered the altitude of Earth-observation SkySats from 500 kilometers to 450 kilometers to improve the resolution of SkySat imagery. Recently, Cognitive Space CEO Scott Herman claimed that “To get higher resolution and still play in a smallsat world, your only option is to go lower” as reported in SpaceNews. Unfortunately, for satellites lacking propulsion, lowering the altitude is not only a lengthy process but will also decrease the overall constellation lifetime, among other potential unwanted effects. Conversely, adding the propulsive capabilities of ATHENA™ allows for lowering the constellation altitude and maintaining it at that higher drag scenario, ensuring the required constellation operational life.
Orbit transfer
For the reasons above, we decided to simulate two additional maneuvers to improve Flock 2p resolution and extend their lifetime. First, we add an “Orbit Transfer” maneuver to perform the altitude lowering, with a target of 450km (decreasing the altitude 60 km from the original injection). The transfer maneuver consists in using in-plane tangential thrust, opposite to the velocity vector, along two arcs centered at the perigee and apogee, to reduce the semi-major axis while preventing an increase in eccentricity.
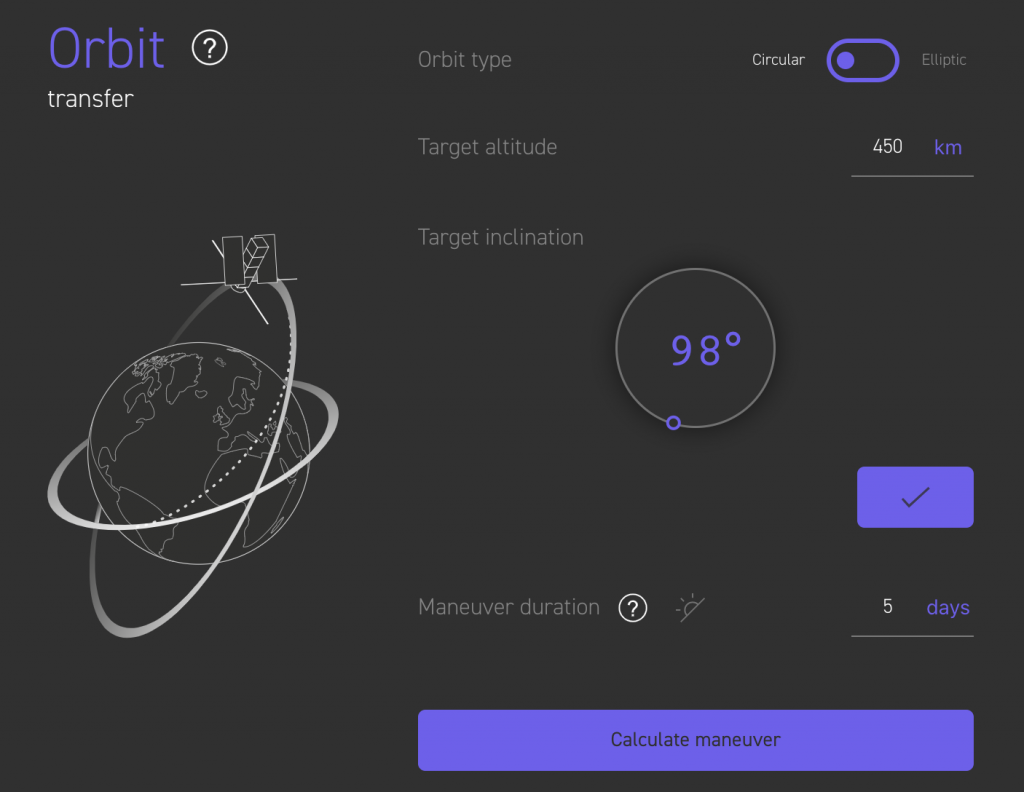
The shortest maneuver (Fig. 8) is found by setting the time of flight as the objective function in GO, which results in thrusting along the entire revolution at maximum power (9 W) for 4.6 days, consuming a total of 10.2 g of propellant. It is also possible to set the propellant mass as objective, to be minimized. However, given the little amount of propellant consumed, and for the purpose of a fast deployment, the shortest maneuver is kept. Once target altitude is reached, the final step is to maintain it for at least the original mission lifetime, and, if possible, longer. We add a third maneuver: “Station keeping”.
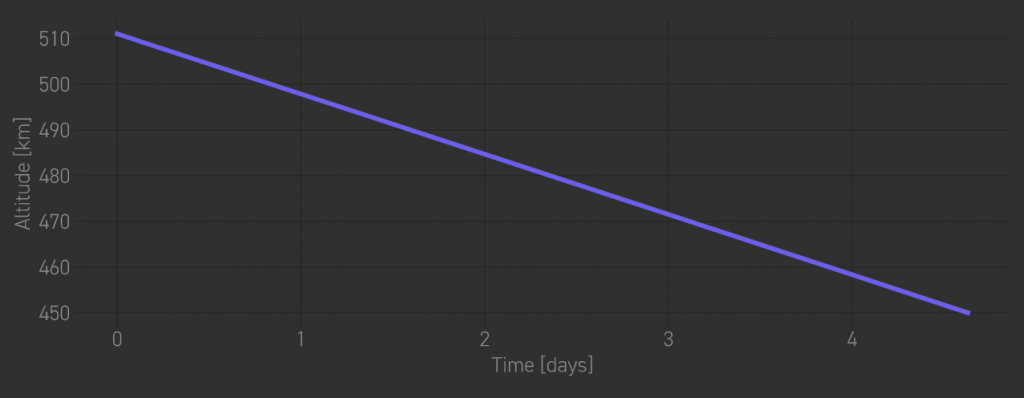
Station keeping
Finally, we also simulate the “Station keeping” maneuver using an altitude tolerance of 3% (~13.5 km) from the nominal orbit. Station keeping in LEO involves mainly preventing the orbital decay of the satellite from the secular effects of atmospheric drag. In this maneuver, we seek to maintain Flock 2p orbit altitude during at least 3.5 years, as before, allowing for a much longer time at the operational orbit than using differential drag.
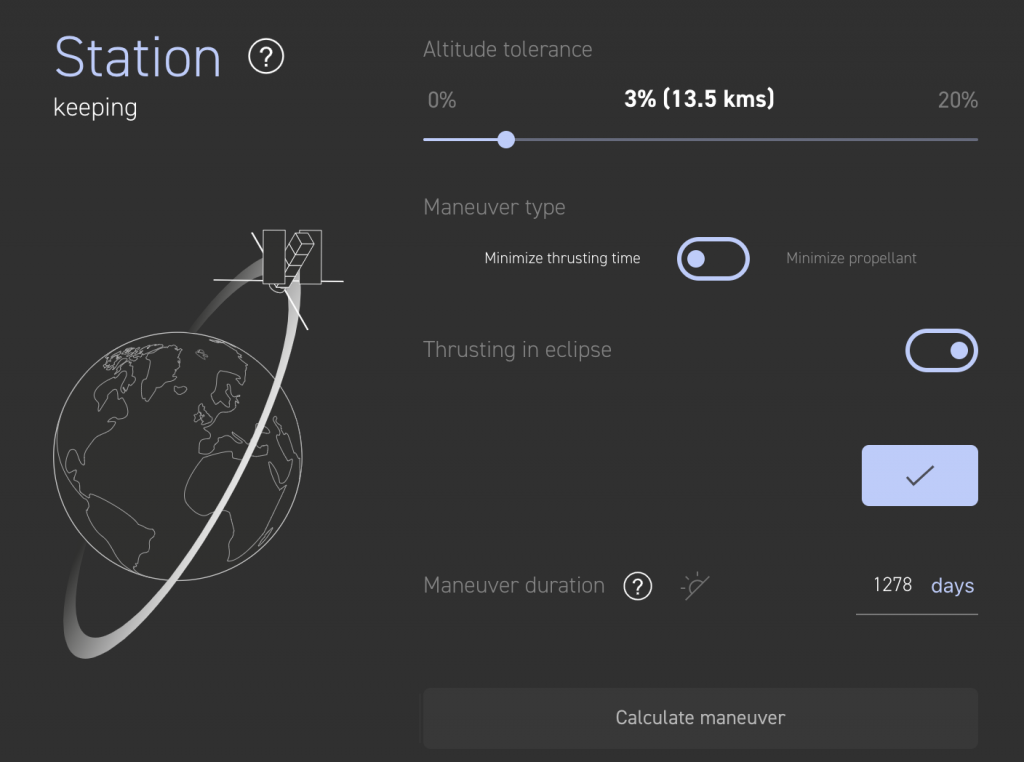
As shown in Fig. 9, the selected time for maneuver duration was 3.5 years, which is already 1 additional year with respect to the nominal value. Additionally, the maneuver type is set to “minimize thrusting time” to reduce the impact of maneuvering on payload operation.
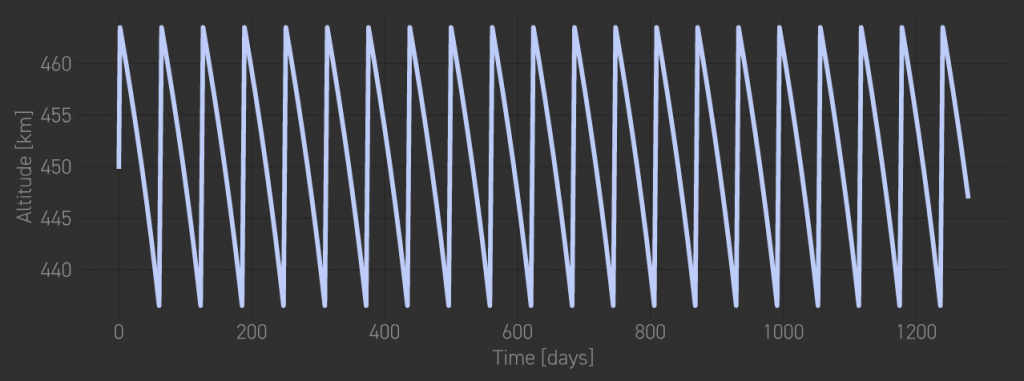
The results are depicted in Fig. 10 and consist of 22 altitude corrections distributed over the required 3.5 years. This maneuver consumes 102.5 g of propellant and takes 46.5 days of thrusting time to be completed. This means that only 3.6% of the entire time was spent actually maneuvering. If we use the previously stated upper limit mass (1 kg of wet mass), the operational altitude could be maintained for more than 10 years.
CONCLUSIONS
The entire thrusting time for study is about 58.2 days as shown in Figure 11, with deployment to the operational orbit achieved after the first 11 days. The propulsion system uses 128.3 grams of propellant, respecting the mass limitation imposed in the mission configuration. Once all maneuvers are calculated, you can click on “Go to Mission Overview” to see a summary of the maneuvers and propulsive requirements. GO™ automatically optimizes and builds a configuration of ATHENA™ that is optimal for the given mission, thus solving the “inverse propulsion problem”. These results are good to go for a theoretical preliminary design phase such as Phase 0/A. In the “Mission Overview” view you may also find the final characteristics of the thruster in terms of power, masses and volume and even export the thruster as a “.step” file to be added to your satellite CAD. Figure 12 shows the mission characteristics aggregated in a visual representation and in table format. You can also download all the data presented in GO™ as a “.pdf” for your records or to share it with your peers.
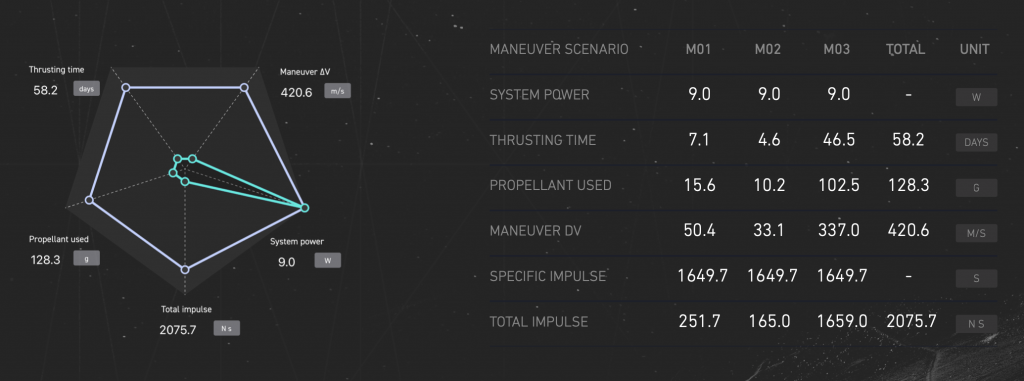
On-board propulsion gives space companies the freedom of selecting different launchers, maneuvering in space, deploying constellations, and safely deorbiting their satellites. In Flock 2p, adding an ATHENA™ thruster would allow for reducing the deployment time of the entire constellation from about 300 days to just 12 days (including altitude reduction), ensuring correct operational capabilities, in terms of coverage, since the start of the mission. Furthermore, the resolution provided by the Earth Observation constellation may be improved, together with faster revisit rates, while still performing station keeping for at least 3.5 years, considerably extending the lifetime of the mission. The operational and performance gains obtained by adding a propulsion system are thus clear and offset the potential cost of adding a custom propulsion system to the satellite. In addition, Figure 12 presents the final configuration of ATHENA™ thruster with 6 thruster heads, the wet mass is less than 16% of the platform mass and the volume fits in less than 0.4U; such small volumes are critical to potentially fit the propulsion system on the already full Dove platform. Furthermore, IENAI SPACE can offer additional customization capabilities for the ATHENA™ thrusters, tuning the form factor in a way that would be more agreeable with the existing subsystems in the spacecraft, without requiring extensive re-engineering.
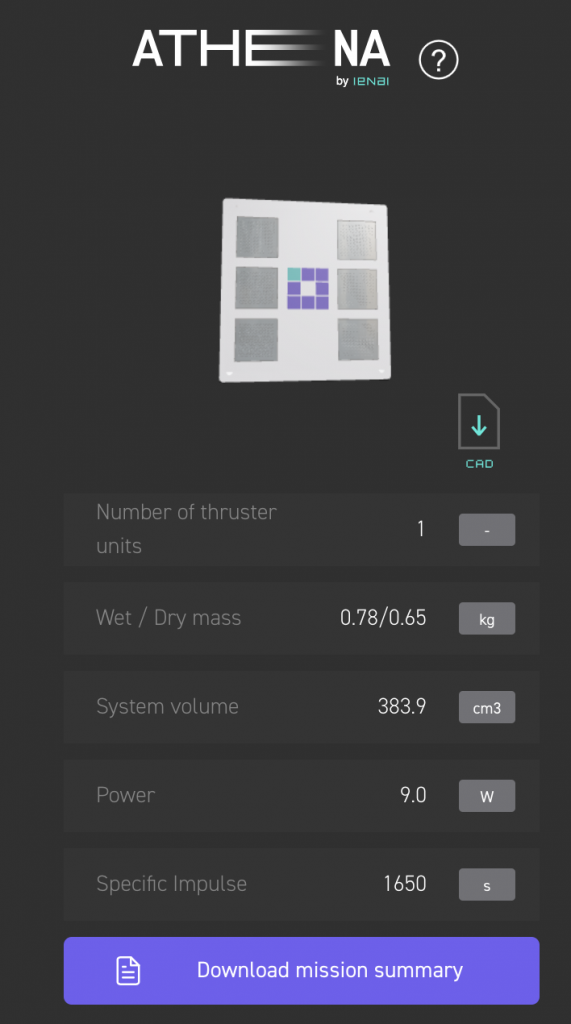
→Download the summary for the Dove mission!
→Register for GO™ and create your custom thrusters!